ABSTRACT
Climate change has caused deserts, already defined by climatic extremes,
to warm and dry more rapidly than other ecoregions in the contiguous United States over the last 50 years. Desert birds persist near the edge of their physiological limits, and climate change could cause lethal dehydration and hyperthermia, leading to decline or extirpation of some species. We evaluated how desert birds have responded to climate and habitat change by resurveying historic sites throughout the Mojave Desert that were originally surveyed for avian diversity during the early 20th century by Joseph Grinnell and colleagues. We found strong evidence of an avian community in collapse. Sites lost on average 43% of their species, and occupancy probability declined significantly for 39 of 135 breeding birds. The common raven was the only native species to substantially increase across survey sites. Climate change, particularly decline in precipitation, was the most important driver of site-level persistence, while habitat change had a secondary influence. Habitat preference and diet were the two most important species traits associated with occupancy change.
The presence of surface water reduced the loss of site-level richness, creating refugia. The collapse of the avian community over the past century may indicate a larger imbalance in the Mojave and provide an early warning of future ecosystem disintegration, given climate models unanimously predict an increasingly dry and hot future.
STATEMENT OF SIGNIFICANCE
Deserts, already defined by climatic extremes, have warmed and dried more than other regions in the contiguous United States due to climate change. Our resurveys of sites originally visited in the early 20th century found Mojave Desert birds strongly declined in occupancy and sites lost nearly half of their species. Declines were associated with climate change, particularly decreased precipitation. The magnitude of the decline in the avian community and the absence of species that were local climatological “winners” are exceptional. Our results provide evidence that bird communities in the Mojave Desert have collapsed to a new, lower baseline. Declines could accelerate with future climate change, as this region is predicted to become drier and hotter by the end of the century.
INTRODUCTION
Species extinctions and population declines have accelerated over recent decades due to habitat destruction, overexploitation, and invasive species (Pimm et al. 2014; Ceballos et al. 2017), with cascading effects on ecosystem functions and services as well as human well-being (Dirzo et al. 2014). Climate change has emerged as another powerful driver of species decline, one whose effects are beginning to intensify. It should lead to shifts in species distributions and rearranged communities (Huang et al. 2017), unless climatic disruption acts as a systemic threat leading to a community collapse (Bergstrom et al. 2015).
Deserts are important bellwethers of climate change. Already defined by climatic extremes, deserts have warmed and dried more rapidly over the last 50 years than other ecoregions, both globally and in the contiguous United States (Zhou et el. 2015; Wuebbles et al. 2017). These trends are predicted to continue through the end of the century (Dominguez et al. 2010). Climate change impacts desert species through the direct effects of thermal and hydric stress, and indirectly via impacts on habitat and food resources. Negative effects of increased temperatures have been documented for desert birds (du Plessis et al. 2012), mammals (Moses et al. 2012), invertebrates (Crawford 1981), and reptiles (Sinervo et al. 2010). Moreover, because precipitation and primary productivity are strongly linked in deserts, drying mediates productivity declines that can permeate across trophic levels through an entire desert community (Chesson et al. 2004)
Desert birds comprise a species-rich, easily detectable assemblage, and are closely coupled to their physical environment, which makes them suitable indicators of climatic change (Wolf 2000). Although desert birds exhibit some adaptive capacity to tolerate thermal and hydric extremes (Williams and Tieleman 2005), many already persist at the edge of their physiological limits (Albright et al. 2017). Both heat waves and the chronic deleterious effects that high temperatures can have on fitness imperil desert birds (Wolf 2000). Furthermore, future warming and associated lethal dehydration risk could extirpate species from the Desert Southwest, particularly small-bodied birds (Albright et al. 2017). Studies of the effects of climate change on North American desert birds are limited (Hargrove and Rotenberry 2011; Cruz-McDonnell and Wolf 2016), but continental-scale surveys suggest arid-land birds are in decline (Sauer 2017). However, the drivers of this decline have not yet been evaluated.
We assessed how climate change and other stressors have impacted desert bird populations over the past century by resurveying sites throughout the Mojave Desert that were originally surveyed for avian diversity in the early 20th century by Joseph Grinnell and colleagues. About 85% of desert lands in this region are largely undisturbed and ecologically intact (Randall et al. 2010), allowing for assessment of the impacts of climate change without the confounding effects of land-use change. Nevertheless, structural changes to habitat induced by grazing and increased severity and frequency of fire are potentially important threats to Mojave birds (Brooks and Pyke 2001). Invasive plants occur in the Mojave, but their primary ecological impact has been to increase fire fuel loads and promote recurrent fires (Brooks and Pyke 2001). Thus, we used fire return intervals as a proxy for the primary impact of invasive plants on habitat; see the Supporting Information (SI) Appendix for a review of invasive plant impacts in the Mojave. We related changes over the past century in occupancy and richness of the Mojave avian community to changes in climate (annual precipitation, average temperature, and maximum temperature), disturbance (fire and grazing), ecosystem productivity facilitated by actual evapotranspiration (AET) and inhibited by climate water deficit (CWD), and recent weather (precipitation, average temperature, and maximum temperature of the previous year) using a dynamic multispecies occupancy model (Dorazio et al. 2010). The analysis quantifies species and community change simultaneously, while accounting for species and survey differences in detectability.
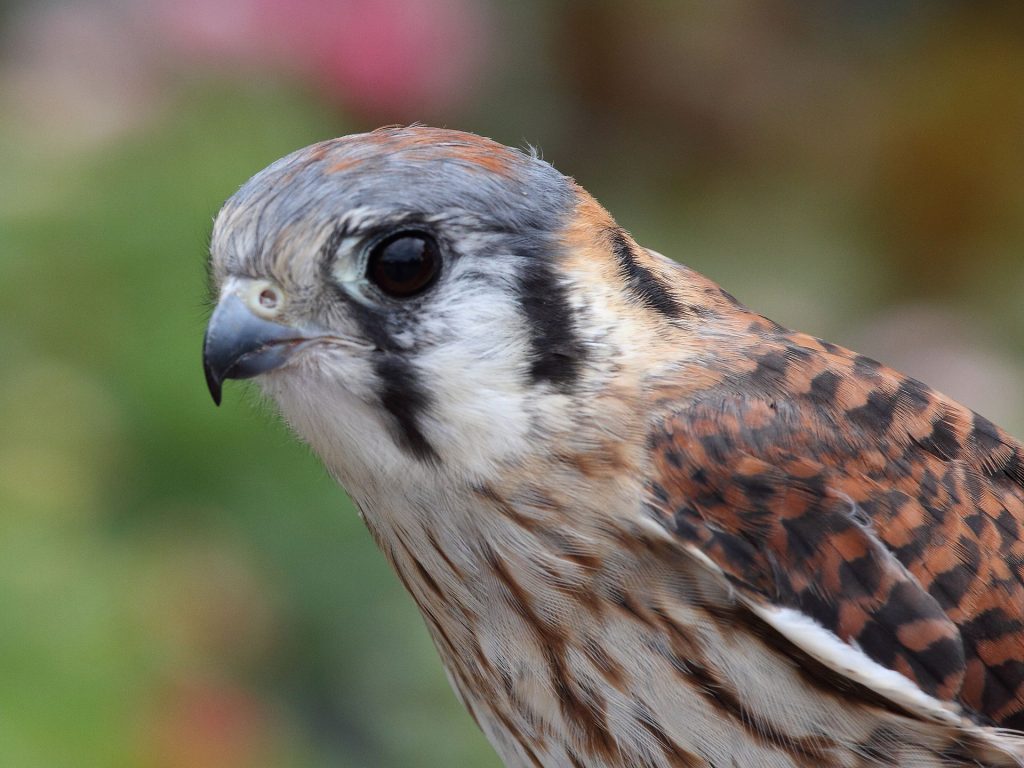
For breeding birds of the Mojave, we examined (i) the relative importance of climate change, habitat change, and recent weather conditions to avifauna change; (ii) if species’ life-history traits or phylogeny accounted for change; and (iii) the persistent characteristics of sites that maintained diversity. We predicted that persistence of the avian community would be negatively related to recent and long-term increases in temperature and disturbance, and positively related to increases in precipitation and habitat productivity. We also predicted that species would experience less decline in occupancy than their counterparts if they had higher mobility, higher dispersal capacity, greater plasticity in resource use, and greater reproductive potential and were better adapted to physiological stress and to desert environments. Additionally, we predicted that sites at higher elevations and latitudes, and those with surface water, would have refugial properties and support higher levels of avian diversity in the modern surveys. Finally, we examined whether the fate of sites varied by management unit to better inform conservation needs. We predicted that sites managed by the US National Park Service (NPS) would fare better than sites on multiuse public lands because of the greater level of protection that park status bestows.
RESULTS
Change in the Mojave avian community over the past century. Change in the Mojave avian community over the past century was characterized by species loss without replacement at resurveyed sites (Figure 1). A strong signal of avifaunal decline is apparent from the community-level measures estimated by the dynamic multispecies occupancy model, for which there was no evidence of lack of fit to the data [posterior predictive check (PPC) = 0.13]. Across the community, the mean probability of species persistence at occupied sites between the two time periods was moderately low [ϕ = 0.43; 95% credible interval (CRI) 0.31 to 0.55], and the mean probability of species colonizing unoccupied sites was close to zero (γ = 0.003; 95% CRI <0.001 to 0.009). Only the common raven and Bewick’s wren had colonization probabilities >0.10. On the other hand, species persistence probabilities at sites were uniformly distributed across the community, with mean values ranging from 0.03 to 0.96. See SI Appendix, Tables S1 and S2, and Dataset S1, for a summary of posterior distributions for community and species values.
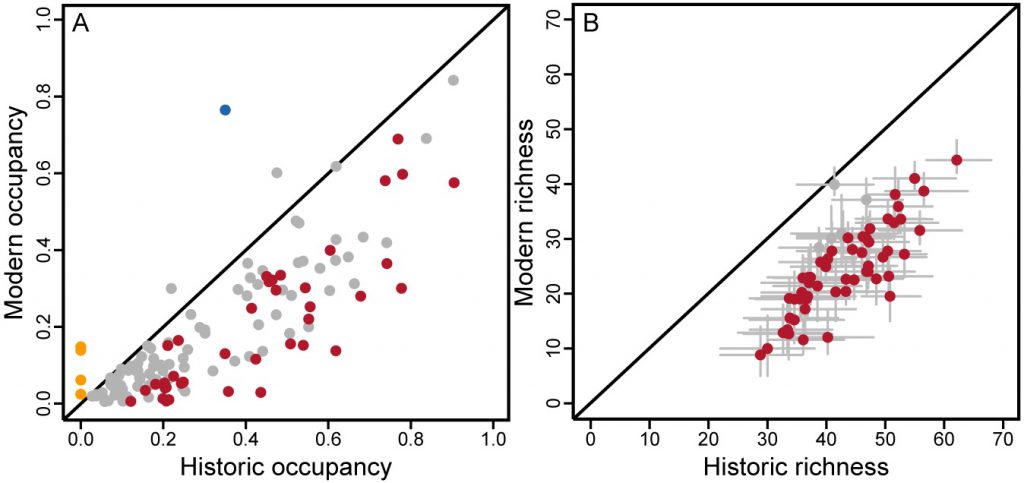
The interplay of colonization and persistence dynamics resulted in an average modern occupancy probability (ψm = 0.11; 95% CRI 0.07 to 0.15) that was significantly lower than historic occupancy (ψh = 0.24; 95% CRI 0.17 to 0.32). Of 135 species, occupancy declined significantly for 39 and increased significantly for only one, the common raven (Figure 1A and Dataset S1). Four species absent historically—the nonnative European starling (EUST), chukar (CHUK), and Eurasian collared-dove (EUCD), and the invasive great-tailed grackle (GTGR)—now occur at a limited number of sites (mean ψm: 0.02 to 0.15). Species with large significant decreases in occupancy probabilities (>0.30) included the American kestrel, northern mockingbird, prairie falcon, turkey vulture, western wood-pewee, white-throated swift, western kingbird, chipping sparrow, mourning dove, sharp-shinned hawk, violet-green swallow, and Brewer’s sparrow. Most were widespread historically (ψh > 0.50). Forty additional species (30%) experienced nonsignificant declines in mean occupancy probability by 0.20 to 0.35 (n = 18) or by 0.10 to 0.20 (n = 22).
Occupancy declines translated into site-level losses of species richness (Figure 1B). Sites decreased on average by 17.9 bird species (95% CRI –20.5 to –15.5), or 42% of their richness, from the historic time period. As a result, 55 of 61 sites experienced a significant decline in richness. This drop in species richness was the result of a mean loss of 20.9 species per site (95% CRI –13.0 to –29.3) and a mean gain of 3.0 species (95% CRI 0.6 to 5.6). The decline in avian occupancy and richness from the historic to the modern era was not due to lower detectability of species during our resurveys. Species present at a site during modern surveys were very likely to be encountered at least once (pm* = 0.94; 95% CRI 0.91 to 0.96), which was significantly higher than during the historic surveys (ph* = 0.57; 95% CRI 0.48 to 0.65).
Drivers of Mojave avifauna change. Decline of Mojave birds over the past century was driven primarily by climate change and secondarily by habitat change (Figure 2; Table 1). A long-term reduction in precipitation best described changes in site-level persistence of Mojave birds and had significant effects for 35 species (Table 1). Maximum temperature and precipitation in the year before resurveys affected community persistence to a lesser degree, describing significant declines of only five and two species, respectively. Moreover, contrary to expectations, community persistence was positively related to maximum temperature in the year before resurveys. Long-term climatic changes of mean and maximum temperature, and mean temperature in the year before resurveys were not important predictors of community persistence. Only one habitat factor was related to community persistence—change in AET, with 14 species responding negatively (Figure 2; Table 1).
Declines in avian occupancy were distributed relatively evenly throughout the avian evolutionary tree (Blomberg’s K, P = 0.45). However, a randomization test found occupancy change clustered in a few families (SI Appendix, Figure S4). Corvidae, Troglodytidae, and Polioptilidae declined significantly less, while Accipitridae and Falconidae declined significantly more than other families.
The two most important predictors of relative occupancy change (ψr) were habitat and diet specialization (Figure 3 and SI Appendix, Table S3). Together they accounted for a total Akaike information criterion weight of 0.89. Arid-land specialists and habitat generalists declined significantly less than grassland and forest species (Figure 3A). Carnivores declined significantly more than insectivores and herbivores, while omnivores declined significantly more than herbivores (Figure 3B). There was less support for effects of range margin: Species with patchy distributions declined significantly more than species without a distributional limit in the study area (Figure 3C). Migratory status, elevational preference, territory type, body mass, and clutch size were poor predictors of decline (SI Appendix, Figure S5).
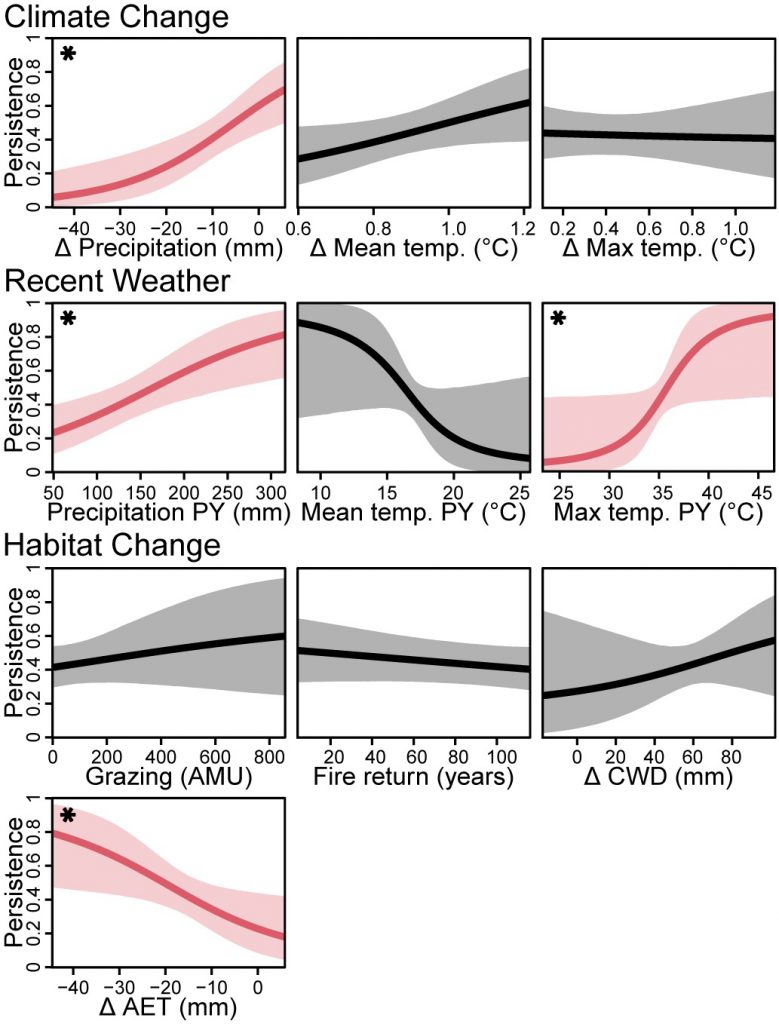
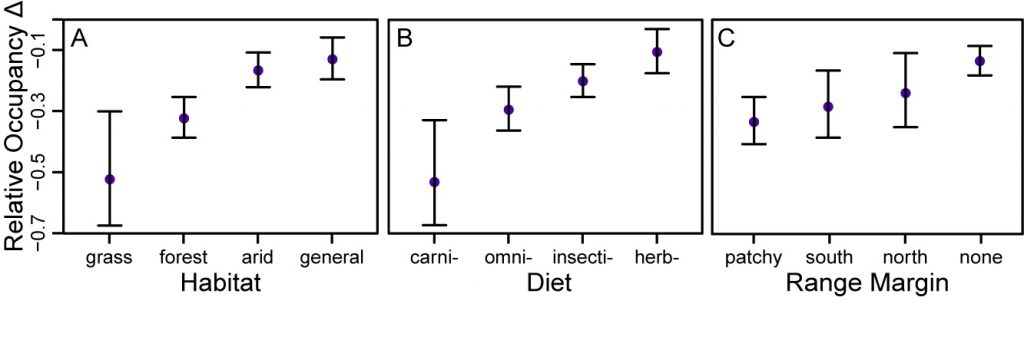
Two key site-level characteristics were associated with reduced rates of species loss for Mojave birds (Figure 4 and SI Appendix, Table S4). The presence of surface water was the most important predictor of relative change in richness (N_r), and sites with surface water present experienced 13.4% less decline than sites without surface water (Figure 4A). Management unit was next most important factor, but protected park lands were not necessarily less immune to species loss than other public lands (Figure 4B). Sites in Death Valley National Park incurred the greatest declines in N_r compared with all other management units, which did not differ (Figure 4B). Higher levels of richness were maintained somewhat better at higher-elevation sites, with 6.3% less decline in N_rfor every 1,000 m in elevation gain (Figure 4C). Latitude had little influence over the geographic scale we investigated (Figure 4D).
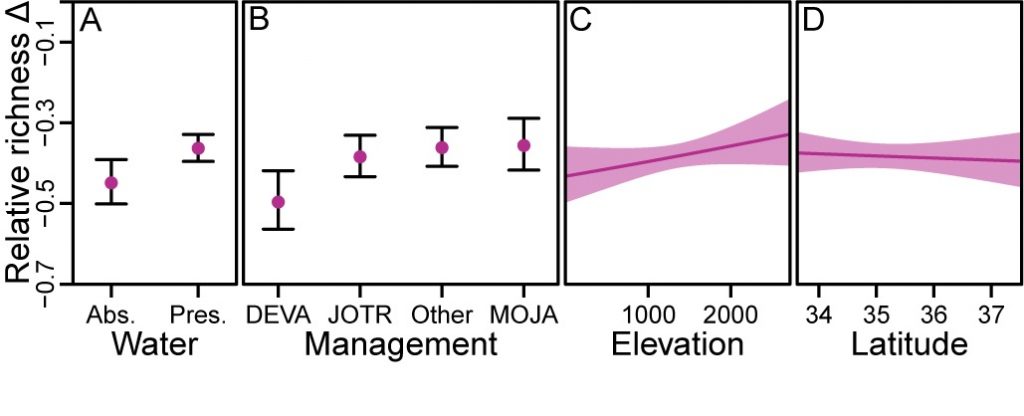
Figure 4. Relationship between site characteristics and relative richness change: (A) the absence/presence of surface water; (B) management unit [Death Valley National Park (DEVA), Joshua Tree National Park (JOTR), and Mojave National Preserve (MOJA)]; (C) elevation (m); and (D) latitude. Error bars and shading represent 95% confidence intervals.
DISCUSSION
Collapse of a desert bird community. Birds of the Mojave Desert exhibited a dominant signal of occupancy decline and erosion of site-level species richness over the last century (Figure 1). Both diminished by about half: the average species occupancy probability declined from 0.24 to 0.11, and sites lost on average 43% of their species. The decline of birds in the Mojave Desert appears to be exceptional compared with resurveys of adjacent ecoregions in the Sierra Nevada (Tingley and Beissinger 2013) and Central Valley (MacLean 2018) that occurred on the same timescale, at similar geographic scales, and following the same methods. Whether in the more diverse Sierras (n = 205 species) or slightly less diverse Central Valley (n = 122), little change in richness occurred over the past century (mean of –2.6 and +1.9 species per site, respectively) compared with the Mojave (n = 135 species) that experienced a loss of 17.9 species per site. Moreover, there were roughly similar increases and decreases in species-level occupancy in the Sierras (10.2% of species decreased and 19.5% increased significantly) and the Central Valley (22.1% decreased and 28.7% increased). In contrast, there was no counterbalance to decline of species (28.9%) in the Mojave, where only the common raven increased significantly in occupancy. Beyond California, studies have found increases or stability in species richness, affiliated with expansions of warm-adapted species or generalists, though studies performed thus far have been biased toward temperate regions (Pearce-Higgins and Green 2014). The trends we observed in the Mojave are corroborated by findings of the North American Breeding Bird Survey (BBS). There has been a 46% decline in the abundance of arid-land indicator species since the onset of BBS surveys in 1968 (North American Bird Conservation Initiative, US Committee 2014; Sauer 2017).
The nearly uniform decrease in site-level richness across the Mojave and the dominant signal of decline in the occupancy suggest a community in the process of collapse. Community or ecosystem collapse is usually the result of multiple causes that create synergistic effects and feedbacks. For example, climate change acted in concert with an emerging disease to threaten global amphibian communities (Price et al. 2014), with herbivory to threaten Arctic avifauna (Ims and Henden 2012), and with a complicated suite of threats to imperil Hawaiian avifauna (Paxton 2016). Nevertheless, climate change alone can mediate the collapse of communities (Bergstrom et al. 2015). Whether the collapse of Mojave birds will continue is unknown, although declines of arid-land birds in the BBS may have abated over the past decade (Sauer 2017). Nevertheless, populations have not recovered to initial levels, nor have they approached the historic baselines that our surveys revealed.
The drivers of collapse. There is little evidence that the major drivers of systemic biodiversity loss—disease, pollution, overexploitation, and habitat destruction—are operating in the Mojave. Invasive plants are in the Mojave, but they currently represent a small amount of cover at most of our sites and across the Mojave as a whole (Abella et al. 2015; SI Appendix). Instead, climate change, particularly decline in rainfall, was the most important driver of avian community dynamics in the Mojave Desert. Sites that received less precipitation in recent decades compared with early in the 20th century had higher local extinction probabilities (Figure 2). Most sites became drier (µ = –3.6%, σ = 5.9%), receiving up to 20% less precipitation. The causal relationship between precipitation and avian population dynamics has many linkages, given the widespread effects precipitation has on arid communities (Chesson et al. 2004). Dry periods can cause desert birds to operate at water deficits due to decreased access to water-rich food resources, which can negatively impact fitness (Smit and McKechnie 2015). Reproduction and survival in many arid-land birds are positively related to precipitation, a response likely mediated through effects on food availability, predation, and nest parasitism in arid environments (Morrison and Bolger 2002; McCreedy and van Riper 2014). Rainfall in the year before resurveys also had a positive relationship with local persistence, but the effect was weaker than the influence of long-term change in precipitation (Table 1). Even though California as a whole experienced a historic drought from 2012 to 2017, the most severe impacts occurred outside of the Mojave Desert (Williams et al. 2015), and in the year before our resurveys rainfall was within normal variation (i.e., within 1 σ of 30-year averages) for all but three sites.
Climate change influenced the collapse of the Mojave bird community primarily through precipitation change rather than temperature change. Long-term warming was not strongly related to avian decline, but there was an influence of maximum temperature in the year previous to resurveys (Table 1). Contrary to our expectations, however, community persistence was positively related to the maximum temperature in the year before resurveys, which could occur for several reasons. First, due to topographic complexity in the Mojave, there was high variability in site-level maximum temperatures in the year before resurveys (µ = 31.8°C; σ = 5.6°C). At high-elevation sites, warming may translate to a longer breeding season, leading to greater reproductive success (Pearce-Higgins et al. 2015). Second, observed temperatures may not yet be hot enough to have biological relevance. The physiological costs of heat avoidance and dissipation behaviors begin to negatively impact fitness of some desert birds when temperature exceeds 35°C (du Plessis et al. 2012; Smit et al. 2016; Pattinson et al. 2017); at temperatures >40°C, evaporative water loss accelerates and puts birds at risk for lethal dehydration (Albright et al. 2016). These thermal limits were exceeded at only 26 and 9% of sites, respectively. Albright et al. (2016) suggested that body size would be an important predictor of decline and extirpation in desert bird communities based on its relationship with heat-related dehydration. Body size, however, was a poor predictor of decline in our study (SI Appendix, Table S3), which also supports
our conclusion that the thermal environment did not appear to be a substantial contributor to Mojave bird declines.
Climate change influenced the collapse of the Mojave bird community primarily through precipitation change rather than temperature change.
Habitat change was less important than climate change in driving avian community collapse. Although fire and grazing can impact birds in arid systems (Bock and Bock 1988), neither strongly influenced avian community response in our study. Only change in AET was related to avian persistence and, contrary to our expectations, it had a negative relationship at the community level and for 14 species (Figure 2 and Table 1). AET is strongly correlated with annual net primary productivity in the Mojave Desert (Lane et al. 1984). Our results imply that some Mojave birds responded negatively to long-term habitat change in the form of vegetation growth. The cause of this response, and whether it is associated with native or nonnative vegetation growth, warrant further investigation. Precipitation is used in calculating AET, and the opposing direction of the community response to these two factors appears conflicting. However, species that responded negatively to a change in AET differed from those species that responded positively to precipitation change. Heterogeneity among species in response to environmental change is relatively common in avian communities (Huang et al. 2017), and is expected given the taxonomic diversity that comprises the Mojave bird community.
Winners and losers. There was an apparent lack of climatological “winners” among the Mojave avifauna. The only species occurring in historic surveys that increased significantly was the common raven, likely due to the proliferation of anthropogenic food resources (Boarman 2003). Relative change in occupancy was randomly dispersed across the phylogenetic tree, with corvids, wrens, and gnatcatchers declining less severely than others (SI Appendix, Figure S4). Arid-land specialists, habitat generalists, and more broadly distributed species were also more resilient to decline, but these groups still decreased, just to a lesser degree (Figure 3). The void left by declining species in many avian communities affected by climate change has been filled, at least partly, by an influx of species for whom the region has become more favorable (Tingley and Beissinger 2013; Pearce-Higgins and Green 2014; Pearce-Higgins et al. 2015; MacLean et al. 2018). While the Sonoran Desert immediately south of the Mojave holds the anticipated pool of more desert-hardy species, we found no expansion or increase of Sonoran-representative birds already present in the study area (e.g., phainopepla, verdin, and black-throated sparrow). Although these absences may represent delays in immigration, birds are a highly mobile taxa and avian communities have responded rapidly to changing climate with lag times as short as 1 to 3 years (Lindström et al. 2013), which suggests that the decline may represent a meaningful change in the community baseline.
Declines in occupancy occurred broadly in Mojave birds, across both common and rare species (Figure 1). Globally, analogous population declines have been identified for one-third of all terrestrial vertebrate species, regardless of rarity (Ceballos et al. 2017). Decline of widespread species is an often overlooked aspect of biodiversity loss that has far-reaching implications for ecosystem function. In the Mojave, carnivores were impacted more severely than other dietary guilds (Figure 3). This trend was also reflected in our family clustering analysis, in which falcons and accipiters experienced significantly greater declines than other families (SI Appendix, Figure S4). This result is in accordance with theoretical and empirical evidence for greater sensitivity of higher trophic levels to climate change, either due to amplification of declines at lower trophic levels or to a temporal mismatch with prey resources (Berggren et al. 2009). Loss of predators has led to trophic downgrading and greatly altered the structure and function in other ecosystems (Whelan et al. 2008).
Conservation implications. Our results provide evidence that bird communities in the Mojave Desert are collapsing to a new, lower baseline that supports about half the local species richness that was present a century ago. The disintegration of avian biodiversity may be an indication of a larger imbalance in the Mojave and an early-warning indicator of future ecosystem collapse. Birds serve many functional roles—predators, pollinators, scavengers, and seed dispersers—and their decline could have cascading effects on ecosystem function and many ecosystem services (Whelan et al. 2008). Moreover, the warming and drying that are unequivocally projected to occur in the Mojave Desert over the next century could further exacerbate or compound the current decline in the avian community (Dominguez et al. 2010). Sites with higher levels of precipitation, with surface water, and at higher elevations (Figure 4) may provide refugia in the face of future climate change. Desert springs across the region are drying from groundwater removal (Patten et al. 2008), and changes in water management policies are needed to reverse this trend. Installation of artificial water sources may mitigate hydric stress and buoy avian diversity, but it is a temporary measure.
Collapse of the Mojave bird community has occurred without regard to the protected status of desert lands. The strongest declines were in the largest national park in the continental United States, Death Valley, where 90% of the land is designated as wilderness. Protected areas are only partly effective in mitigating population declines caused by the broad-reaching consequences of human activities (Barnes et al. 2016), and combating climate change will require global policy efforts in addition to local actions to reduce greenhouse gas emissions. The Mojave, though relatively undeveloped, is experiencing considerable pressure from the renewable energy sector (Cameron et al. 2012). Protecting biodiversity while reducing dependence on fossil fuels will require smart development of green energy.
Community collapse of the Mojave avifauna represents a precursor to defaunation that would have been overlooked if we had not had access to the original faunal surveys of Joseph Grinnell and colleagues from the early 20th century. Data used to document declining wildlife populations typically trace to the 1960s or 1970s (Dirzo et al. 2014; Bonebrake et al. 2010). Although similar changes may be occurring in other ecoregions that lack comparable historical data, the harsh nature of desert environments makes them more likely to become less suitable for life and offers a prescient warning for biodiversity loss as future climates are pushed further toward extremes.
MATERIALS AND METHODS
Bird surveys. Surveys of avian diversity were performed at 61 sites across the Mojave Desert spanning a broad latitudinal (33.6° to 37.5°), longitudinal (–117.7° to –114.8°), and elevational range (–17.8 to 3396.2 m) (SI Appendix, Figure S1). The majority of sites (58 of 61) were on federal lands managed by NPS, US Bureau of Land Management (BLM), US Forest Service (USFS), and US Fish and Wildlife Service (SI Appendix, Figure S1). Only one site experienced development from the historic time period; resurvey of this site occurred <1 km away in habitat matching historic descriptions.
Historic surveys were conducted between 1908 and 1968, with 70% of the surveys occurring between 1917 and 1947. Historic survey data (species encounters, geographic locations, and metadata) were extracted from field notes archived at the Museum of Vertebrate Zoology, University of California, Berkeley. Historic surveys followed a precursor of the line-transect method and provided a record of detection/nondetection data for all species encountered.
Historic data at each site were restricted to surveys that occurred within the breeding season of a single year to ensure closure during the primary sampling period. To reduce bias that a small number of heavily sampled sites might have on estimated detection probabilities, we limited historic data to ≤10 surveys within a single year. Seven historic surveys followed a removal protocol (MacKenzie et al. 2006), and were analyzed
as such.
Modern resurveys occurred between 2013 and 2016 and followed standard avian variable-distance point count methods and protocols established by the Grinnell Resurvey Project (Tingley and Beissinger 2013). Transects consisted of 10 sampling points separated by >250 m that spanned the area, elevational extent, and vegetation heterogeneity of historic survey routes. Bird counts from modern surveys were reduced to detection/nondetection data per site for comparison with historic data. Six sites surveyed in the modern time period had no historic counterpart, but provide useful information for detection and distribution. See SI Appendix for additional description of protocols.
The historic dataset encompassed 204 surveys at 55 sites, with a mean of 3.46 surveys per site. The modern data included 183 surveys at 61 sites, with 3 surveys per site. A total of 135 breeding species were included in the analysis. Potential for breeding was determined by overlap of expert-defined breeding ranges (50) with survey sites. Northbound migrants (n = 26) and vagrant species (n = 9) were excluded. Nocturnal birds and obligate waterbirds were not adequately surveyed by our methods and were excluded from analysis. Species were analyzed using a revised taxonomic treatment. Four species (EUCD, EUST, CHUK, and GTGR) known to colonize the Mojave after the completion of historic surveys (Small 1994) were treated as being truly absent from the historic data.
Occupancy model for community dynamics. A dynamic multispecies occupancy model (Dorazio et al. 2010) was used to explicitly model the persistence and colonization dynamics that occurred between the historic and modern time periods. Survey data were assumed to be the result of imperfect observation of the true incidence of a species at a site. Occupancy dynamics were modeled as a first-order Markovian process (i.e., incidence of a species in the modern period was assumed to depend on the incidence state of the historic period). Covariates were included as a linear combination of effects with a logit-link transformation for each of the four probabilities estimated by the model [detection (p), historic occupancy (ψ), local persistence (ϕ), and local colonization (γ)]. The species-specific intercept and coefficient values were governed by normal distributions, which were in turn drawn from community-level hyperdistributions. The hyperdistributions for the intercept terms and the mean of the coefficients were assigned vague priors.
We fit the model with a weakly-informative prior applied to the variance of the coefficient hyperdistributions, which behaves as a type of regularization (Hooten and Hobbs 2015). Regularization limits the effects of collinearity in the covariates and prevents overfitting, allowing us to evaluate a global model of community response (Hooten and Hobbs 2015). For full model and prior specification, see SI Appendix.
Model covariates. To evaluate community dynamics between time periods, we fit covariates to each probability modeled. Survey era was included as a categorical covariate of detection to explicitly capture differences between the two time periods (Dorazio et al. 2010). Julian day and its quadratic term were included in the detection model to allow for detectability to vary during the breeding season. Initial occupancy covariates included elevation and its quadratic term, and historic climate averages (annual precipitation, mean annual temperature, and maximum annual temperature). Since the model-estimated mean colonization probability was very low (γ = 0.003; 95% CRI <0.001 to 0.009), we only included one nuisance covariate on colonization to avoid overfitting—years elapsed between historic and modern surveys. This covariate was also included for persistence.
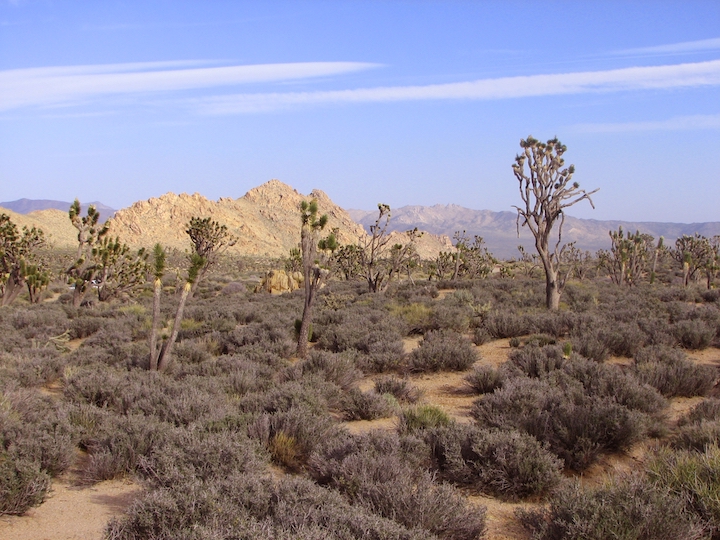
Covariates chosen to assess the drivers of community persistence fell into three categories: climatic change (annual precipitation change, annual mean temperature change, annual maximum temperature change), recent weather (precipitation, mean temperature and maximum temperature of the year before the modern surveys), and habitat change (grazing intensity, mean fire return interval, CWD change, AET change). Vegetation descriptions from the historic surveys were qualitative in nature, so we were unable to evaluate effects of fine-scale habitat change on community dynamics, including the potential effects of invasive plant species beyond their impact on altered fire regimes (SI Appendix). Environmental covariates were calculated from the Basin Characterization Model (BCM: Flint et al. 2013), using a 5-km window to capture local variability. Change covariates were calculated from differences between historic climate (1906 to 1965) and modern (1986 to 2015) climate averages. Historic climate averages and weather of the previous year were also extracted from the BCM. Mean fire return interval for each site was calculated from fire perimeter spatial databases of Cal Fire’s Fire and Resource Assessment Program (https://frap.fire.ca.gov/) and the Nevada BLM. Current grazing intensity data [animal unit month (AUM) per ha] were provided by NPS, USFS, and BLM regional offices. All covariates were centered and scaled before inclusion.
Model data were preprocessed in R version v3.4.0 (R Core Team 2017) and fit using Markov chain Monte Carlo as implemented by JAGS v4.3.0 (Plummer 2017). We retained 13,000 samples of the posterior (40 chains; burn-in: 10,000 iterations; thinning: 100 iterations). Chains were considered converged when the Gelman-Rubin statistic was ≤1.1 for all stochastic nodes. We used a PPC to test goodness of fit (see SI Appendix for details). We use “significant” to describe effects that have a high probability of being nonzero (i.e., 95% CRIs nonoverlapping with zero), or to describe two values that have a high probability of being different (i.e., nonoverlapping 95% CRIs).
Species traits and site characteristics as predictors of change. Species-specific traits were evaluated for their potential to account for occupancy change: dietary breadth, fecundity, elevational preference, range margin, dispersal ability, body size, and territoriality (SI Appendix, Table S5). We tested the importance of traits as predictors of relative occupancy change (ψr= [ψm– ψh] / ψh) between historic (ψh) and modern (ψm ) surveys using weighted linear regressions. To account for uncertainty in estimates, weights were calculated from the inverse variance of the posterior distribution of ψr, giving higher weight to estimates with lower uncertainty (Hedges et al. 1999). We transformed the data (log[1 + ψr]) to linearize the ratio for both increases and decreases in occupancy (Hedges et al. 1999) and added 0.016, the equivalent of one site being occupied, to occupancy probabilities to adjust for zero estimates that occurred for some species in either time period.
Phylogenetic signal was evaluated to ascertain the independence of species and to identify taxonomic clades that experienced similar responses. We downloaded 500 trees from birdtree.org, 250 of each of the Ericson and the Hackett backbones (Jetz et al. 2014). We calculated Blomberg’s K for all 500 trees to test whether a phylogenetic signal in ψr was present across the evolutionary tree (Blomberg et al. 2003; Frishkoff et al. 2014). We also examined whether response was clustered within taxonomic families, as defined by the American Ornithological Society, through a randomization test; ψr was calculated for each family and compared with 10,000 random draws of the same number of species as in the family (Frishkoff et al. 2014). Species introduced after the historic surveys were excluded from the phylogenetic and trait analyses because their occupancy change was assumed to be related to invasion biology.
Species richness was calculated for each posterior sample as the sum of the species incident at the site during each time period. Site characteristics (elevation, latitude, management unit, and presence of surface water) were tested as predictors of relative change in richness (Nr= [Nm– Nh] / Nh) using weighted linear regressions in the same fashion as ψr.
ACKNOWLEDGMENTS
B O. Wolf, P. Unitt, L. Hargrove, and M.W. Tingley provided valuable comments on the manuscript. L.J. Barth, T.S. Baxter, R.A. Klotz, and C.N. Hawk assisted with bird surveys. This research was performed with support from the National Science Foundation (DEB-1457742, DEB-1501757, DGE-1106400), National Geographic Society, NPS, and Museum of Vertebrate Zoology and Department of Environmental Science, Policy, and Management at the University of California, Berkeley.
REFERENCES
Abella, S., et al. 2015. Status and management of non-native plant invasion in three of the largest national parks in the United States. Nature Conservation 10: 71–94.
Albright, T.P., et al. 2017. Mapping evaporative water loss in desert passerines reveals an expanding threat of lethal dehydration. Proceedings of the National Academy of Sciences 114(9): 2283–2288.
Barnes, M.D., et al. 2016. Wildlife population trends in protected areas predicted by national socio-economic metrics and body size. Nature Communications 7: 12747.
Berggren, Å., C. Björkman, H. Bylund, and M.P. Ayres. 2009. The distribution and abundance of animal populations in a climate of uncertainty. Oikos 118(8): 1121–1126.
Bergstrom, D.M., et al. 2015. Rapid collapse of a sub-Antarctic alpine ecosystem: The role of climate and pathogens. Journal of Applied Ecology 52(3): 774–783.
Blomberg, S.P., T. Garland, A.R. Ives, and B. Crespi. 2003. Testing for phylogenetic signal in comparative data: Behavioral traits are more labile. Evolution 57(4): 717–745.
Boarman, W.I. 2003. Managing a subsidized predator population: Reducing common raven predation on desert tortoises. Environmental Management 32(2): 205–217.
Bock, C.E., and J.H. Bock. 1988. Grassland birds in southeastern Arizona: Impacts of fire, grazing, and alien vegetation. ICBP Technical Publication 7: 43–58.
Bonebrake, T.C., J. Christensen, C.L. Boggs, and P.R. Ehrlich. 2010. Population decline assessment, historical baselines, and conservation. Conservation Letters 3(6): 371–378.
Brooks, M.L., and D.A. Pyke. 2001. Invasive plants and fire in the deserts of North America. In Proceedings of the Invasive Species Workshop: The Role of Fire in the Control and Spread of Invasive Species, K.EM. Galley and T.P. Wilson, eds. First National Congress on Fire Ecology, Prevention, and Management. Tallahassee, FL: Tall Timbers Research Station, 1–14.
Cameron, D.R., B.S. Cohen, and S.A. Morrison. 2012. An approach to enhance the conservation-compatibility of solar energy development. PLoS ONE 7(6): e38437.
Ceballos, G., P.R. Ehrlich, and R. Dirzo. 2017. Biological annihilation via the ongoing sixth mass extinction signaled by vertebrate population losses and declines. PNAS: 201704949.
Chesson, P., et al. 2004. Resource pulses, species interactions, and diversity maintenance in arid and semi-arid environments. Oecologia 141(2): 236–253.
Crawford, C.S. 1981. Biology of Desert Invertebrates. New York: Springer-Verlag.
Cruz-McDonnell, K.K., and B.O. Wolf. 2016. Rapid warming and drought negatively impact population size and reproductive dynamics of an avian predator in the arid Southwest. Global Change Biology 22(1): 237–253.
Dirzo, R., et al. 2014. Defaunation in the Anthropocene. Science 345(6195): 401–406.
Dorazio, R.M., M. Kéry, J.A. Royle, and M. Plattner. 2010. Models for inference in dynamic metacommunity systems. Ecology 91(8): 2466–2475.
Dominguez, F., J. Cañon, and J. Valdes. 2010. IPCC-AR4 climate simulations for the Southwestern US: The importance of future ENSO projections. Climatic Change 99(3): 499–514.
du Plessis, K.L., R.O. Martin, P.A.R. Hockey, S.J. Cunningham, and A.R. Ridley. 2012. The costs of keeping cool in a warming world: Implications of high temperatures for foraging, thermoregulation and body condition of an arid-zone bird. Global Change Biology 18(10): 3063–3070.
Flint, L.E., A.L. Flint, J.H. Thorne, and R. Boynton. 2013. Fine-scale hydrologic modeling for regional landscape applications: The California Basin Characterization Model development and performance. Ecological Processes 2(1): 1–21.
Frishkoff, L.O., et al. 2014. Loss of avian phylogenetic diversity in Neotropical agricultural systems. Science 345(6202): 1343–1346.
Hargrove, L., and J.T. Rotenberry. 2011. Breeding success at the range margin of a desert species: Implications for a climate-induced elevational shift. Oikos 120(10): 1568–1576.
Hedges, L.V., J. Gurevitch, and P.S. Curtis. 1999. The meta-analysis of response ratios in experimental ecology. Ecology 80(4): 1150–1156.
Hooten, M.B., and N.T. Hobbs. 2015. A guide to Bayesian model selection for ecologists. Ecological Monographs 85(1): 3–28.
Huang, Q., J.R. Sauer, and R.O. Dubayah. 2017. Multidirectional abundance shifts among North American birds and the relative influence of multifaceted climate factors. Global Change Biology 23(9): 3610–3622.
Ims, R.A., and J.A. Henden. 2012. Collapse of an Arctic bird community resulting from ungulate-induced loss of erect shrubs. Biological Conservation 149(1): 2–5.
Jetz, W., et al. 2014. Global distribution and conservation of evolutionary distinctness in birds. Current Biology 24(9): 919–930.
Lane, L.J., E.M. Romney, and T.E. Hakonson. 1984. Water balance calculations and net production of perennial vegetation in the northern Mojave Desert. Journal of Range Management 37(1): 12–18.
Lindström, Å., M. Green, G. Paulson, H.G. Smithm and V. Devictor. 2013. Rapid changes in bird community composition at multiple temporal and spatial scales in response to recent climate change. Ecography 36(3): 313–322.
MacKenzie, D.I., et al. 2006. Occupancy Estimation and Modeling: Inferring Patterns and Dynamics of Species Occurrence. Burlington, MA: Academic Press.
MacLean, S.A. 2018. A century of change in avifauna of California’s most transformed landscapes. Ph.D. dissertation, University of California, Berkeley, Berkeley.
MacLean, S.A., A.F.R. Dominguez, P. de Valpine, and S.R. Beissinger. 2018. A century of climate and land-use change cause species turnover without loss of beta diversity in California’s Central Valley. Global Change Biology 24(12): 5882–5894.
McCreedy, C., and C. van Riper. 2014. Drought-caused delay in nesting of Sonoran Desert birds and its facilitation of parasite- and predator-mediated variation in reproductive success. The Auk 132(1): 235–247.
Morrison, S.A., and D.T. Bolger. 2002. Variation in a sparrow’s reproductive success with rainfall: Food and predator-mediated processes. Oecologia 133(3): 315–324.
Moses, M.R., J.K. Frey, and G.W. Roemer. 2012. Elevated surface temperature depresses survival of banner-tailed kangaroo rats: Will climate change cook a desert icon? Oecologia 168(1): 257–268.
North American Bird Conservation Initiative, US Committee. 2014. The State of the Birds 2014 Report. Washington, DC: US Department of the Interior. Online at http: //www.stateofthebirds.org/2014/. Accessed January 1, 2018.